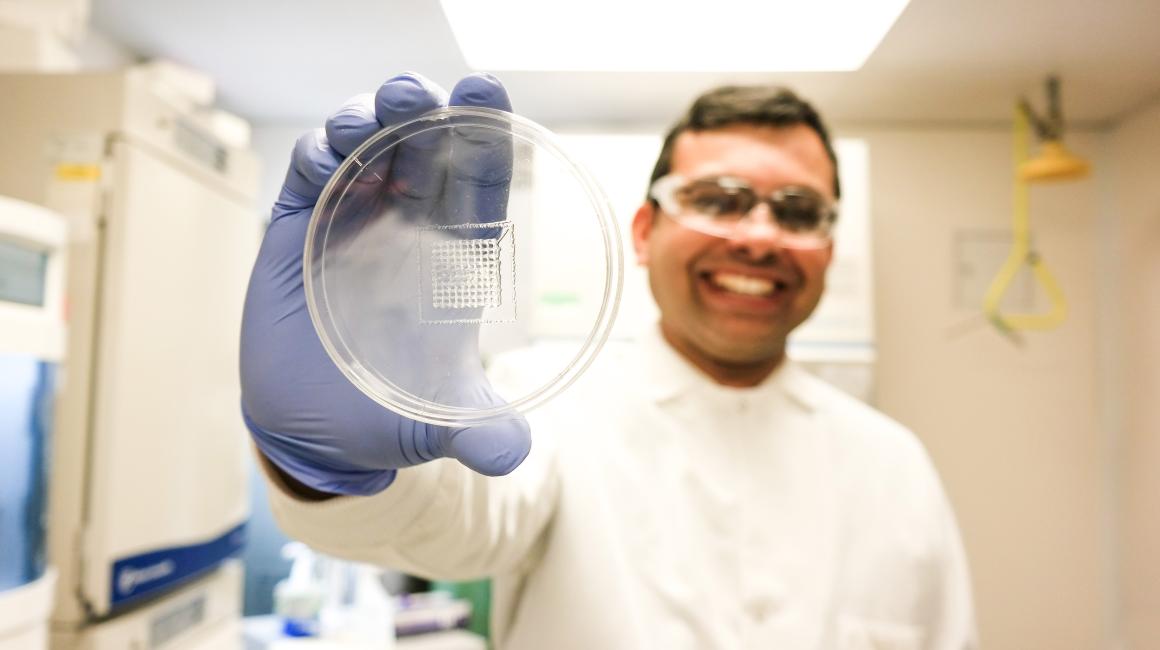
This article was originally published on March 15, 2020.
In Nilay Chakraborty’s lab, the inspiration often comes from surprising places. Take, for example, one of the mechanical engineering associate professor’s recent muses: The tardigrade, a chubby half-millimeter creature that’s happily at home in the so-ugly-it’s-cute category and is considered by scientists to be one of the most indestructible animals on earth. They can be found living in Antarctica, lava fields and most of the planet’s most punishing environments. In extremely dry conditions, they can fully dehydrate, shut down their metabolisms and then wake back up a decade later as if nothing happened. Recently, some tardigrades aboard an Israeli space capsule crash landed on the moon. Scientists say it’s perfectly reasonable to think they survived the impact; previous experiments have already demonstrated tardigrades can survive the radiation and extreme temperatures of outer space.
Chakraborty’s fascination with the tiny creatures began with a problem that presumably had nothing to do with them. One of his main areas of research is human cell preservation — a practice that’s an indispensable part of medical research and clinical practice alike. Biological scientists depend on preserving cells for doing all kinds of experiments, and doctors use it routinely in things like fertility treatments. But despite its importance, Chakraborty says cell preservation, and the basic technique for doing it, hasn’t changed much in the past 40 years. The base technology is still cryogenics — a process of stabilizing cells using chemicals and super low temperatures. It’s effective, with one major caveat: The “antifreeze” compounds needed to protect cells from damage by ice crystals happen to be pretty toxic to living things. “Something like dimethyl sulfoxide — you wouldn't want that anywhere near your body,” Chakraborty says. “You wouldn't even want to put that in your car.”
Scientists have learned to cope with the undesirable side effects of this toxicity, which can include relatively high rates of physical and DNA-level cellular damage. But researchers have consistently been on the hunt for better techniques. That’s how Chakraborty eventually found himself enamored with a creature known for its resurrection abilities. “As an engineer, I have great respect for nature — there really is no better designer. Living things are extremely complex organic machines that got optimized in such a beautiful way over a long period of time. Tardigrades seem to have harnessed nature’s way of preserving cells."

Chakraborty learned that tardigrades’ durability is rooted in special compounds found in their bodies. Under extreme conditions, when they need to go into a suspended state, tardigrades can use these compounds to essentially harden into a glasslike substance. This protects their cells from damage until more favorable conditions allow them to reanimate. The process, in fact, has some fundamental similarities to cryogenic freezing — a fact that inspired Chakraborty to experiment with a cell preservation technique based on the tardigrade's magic chemistry. For his first set of experiments, he essentially diluted a typical cryogenic solution with a solution containing the tardigrade’s special compounds, hoping it would prove less toxic. When he looked closely at the preserved cells, he discovered a glasslike substance was in fact helping protect them against typical damage from ice crystals. Even more importantly, when he woke the cells back up, nearly all of them looked and functioned as if they’d never been frozen in the first place.
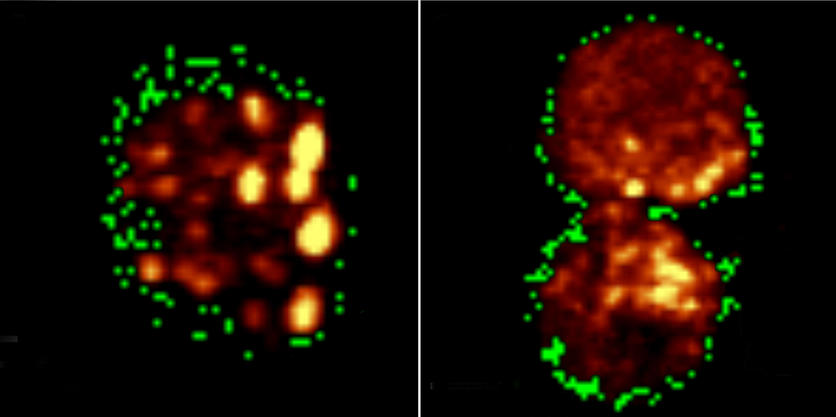
He continued to refine his new approach, zeroing in on the optimal solution, speed and temperature for his preservation process. But he was still bothered by one fact: His improved technique was still dependent on traditional cryogenic technology. To him, this fundamentally limited the scope of its application. He was thinking in particular about potentially revolutionary new treatments like cell therapy, which involve implanting, grafting or injecting lab-grown cells into a patient’s body. But as something requiring preserved cells, cell therapy is fundamentally limited by the current cryogenic paradigm. He says especially when you think about treating patients in rural hospitals or less-developed countries that lack access to cryogenic technology, being dependent on current processes likely means denying a promising new treatment to millions of people.
Ever the engineer, he began thinking about ways to achieve the same results but with a simpler, cheaper process that translated well to clinical applications. That eventually led Chakraborty to entertain what’s arguably his boldest idea to date: To figure out a way to preserve cells using his glasslike substance, but without any cryogenics at all. When he set about doing that, he again approached it like an industryman, looking at other manufacturing processes where thin protective layers had to cure very quickly at relatively normal temperatures. He ran across things like “thin coating,” which lays down thin, sometimes, nano-level protective veneers on everything from semiconductors to LED screens — without the need for extreme heat or cold. This inspired him to then design an experiment that he says works kind of like old-fashioned candle making. With the cells attached to a column-shaped substrate, he could dip the cells in a version of his special solution, pull them out, and then blow a little air over them to encourage the curing of a very thin protective organic glass. The best part: All of this would happen at room temperature — not at the negative 150 degrees Celsius typically required for cryogenic freezing.
That was the idea anyway. But the wildest twist in Chakraborty’s story is that the fairly straightforward process worked like a charm. As before, when he woke his preserved cells back up, they showed completely normal function.
“It really was quite incredible, and we’re very excited about the potential of this method to help speed up all kinds of medical research and treatments,” Chakraborty says. “If you look at biological or clinical practice, there are so many instances where what we’re doing is the same thing we did 30 or 40 years ago. On the other hand, there’s basically a news story every hour about some incredible medical advance that’s going to change everything. So what explains that disconnect? I think one of the really big reasons this happens is we work on things in isolation, and then try to fit our discoveries into a solution model, rather than coming at it from the other side. Engineers — we are always thinking about application. We think about things — even living things — as shaped by the universe’s fundamental properties. So I’m very optimistic that if we start translating our research problems in this way, engineering can have a huge impact on the future of medicine.”