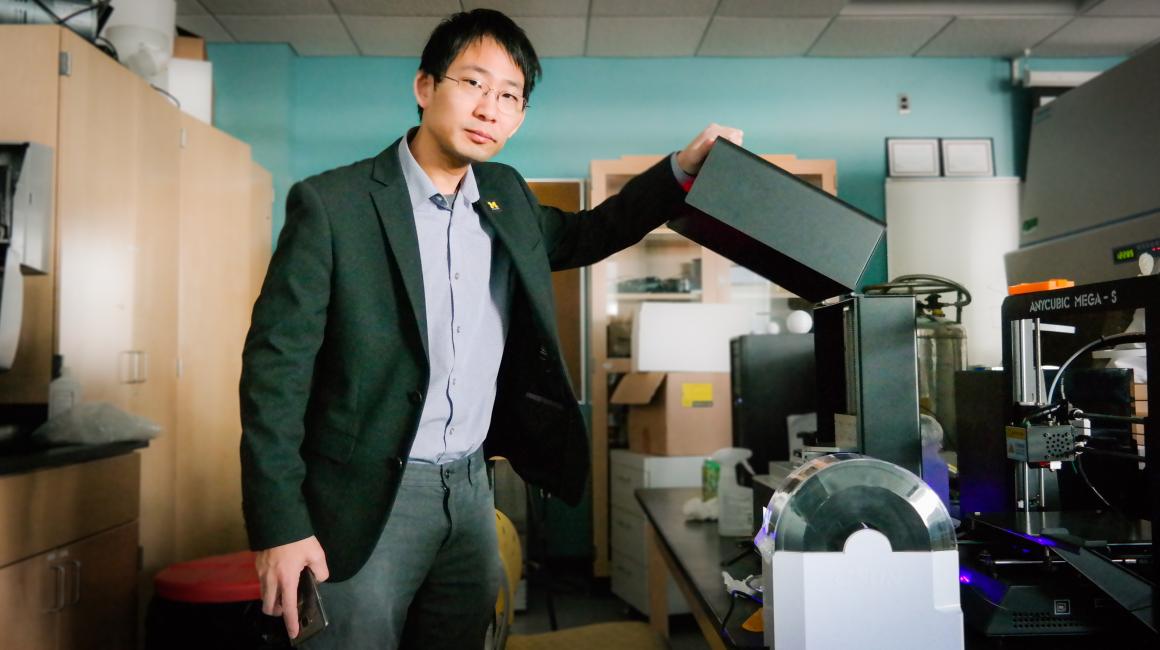
The last time we visited Associate Professor Joe Lo’s lab, he was busy hacking a military-grade night vision scope he bought off eBay and turning it into a high-powered, low-cost device for detecting skin cancers. But his latest research project may even beat that one for creativity. This time, the subject of the hack is a long-forgotten technology from early 20th-century inventor and cult science hero Nikola Tesla. The objective: redeploying it to study one of the fundamental mysteries of diabetes.
So how do we get from an inventor best known for his work on electricity and rivalry with Thomas Edison to a blood sugar disorder that impacts more than 30 million Americans? Lo has actually been building a fascinating body of work around diabetes for years, and as a person who’s schooled in things like fluid dynamics, he’s always been intrigued by the mechanical processes by which the disease exacts its toll on the body. Type II diabetes, of course, has a range of complications, many of which stem from damage to our tiniest nerves and blood vessels. But how the disease, which results from the body’s inability to metabolize glucose efficiency, translates into this kind of damage is still something scientists don’t really understand.
When Lo began searching the medical literature for a way to approach the problem in his lab, he ran across an interesting new finding: Some diabetic patients appear to have more viscous blood. That intrigued Lo since much of the damage from Type II happens in very tiny blood vessels. Could one factor, he thought, be that thick blood was having a harder time traveling into these tiny vessels, causing stress, and eventually damage, to surrounding tissues?
To get started, Lo zeroed in on one particularly fascinating phenomena of Type II diabetes: The fact that Type II diabetics often eventually develop Type I diabetes. With Type II, the body has problems metabolizing glucose because its natural hormone for doing that, insulin, loses its effectiveness. In Type I, the body loses its ability to produce insulin altogether because the cells that make it — the beta cells in the pancreas — get damaged. In Type I diabetics, this is typically due to an autoimmune reaction in which the body attacks its own cells. But in Type IIs, this occurs later in the course of their disease, without an autoimmune reaction. So how exactly are the beta cells getting damaged?
Lo was curious to see if more viscous blood could be one of the factors.
“Pancreatic beta cells in the body are very well vascularized, so they have lots of little capillaries going through them,” Lo explains. “You can understand why — they have to be able to respond directly to the blood to know what glucose levels are. But if it’s true that what I’m calling a ‘pathological viscosity’ of diabetes affects capillaries, then it could definitely have an impact on these beta cells.”
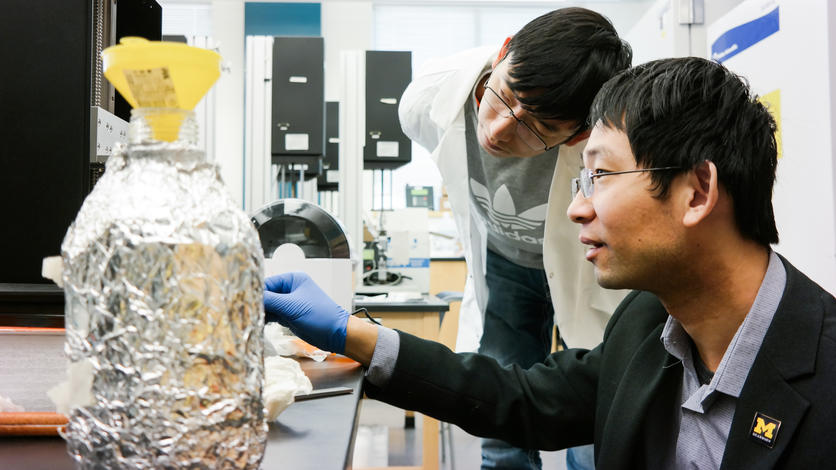
The next challenge, of course, was finding a way to study that — given that direct observation of someone’s tiny pancreatic capillaries in action would be pretty difficult. So Lo began brainstorming a way to recreate a lab model that was capable of simulating the flow of these tiny systems. Straightaway, he ran into a big challenge. In the body, the heart is the main way blood gets pumped to our various organs and tissues. And its forceful beat creates all kinds of pressure gradients as it pulses strongly, then relaxes. But by the time blood reaches tiny capillaries, an interesting thing happens: The heart’s pumping force largely dissipates and this turbulence disappears. In the tiniest capillaries, blood cells actually move in a really smooth, slow, orderly fashion.
You’d think this might make it easier to recreate in a lab, but the opposite is true. It turns out, almost all artificial pumps use bladed turbines to move liquids, and like the heart, these blades create a lot of turbulence. It’s the opposite of the even, “laminar,” “nonpulsatile” flow Lo was looking for.
“So I Google ‘laminar flow’ and ‘nonpulsatile’ flow, and the funny thing is I get back all these results that mention Tesla,” Lo explains. “And it was 2016 — Tesla, the car company, was pushing out the Model III. So I was like, well, OK, none of this is relevant. But then I look closely, and there were all these references to a 1913 patent by Nikola Tesla. It was this invention that involved a big circular stack of disks that Tesla wanted to use to make electric power generation more efficient. And the big thing with these ‘Tesla rotors’ is that they don’t create the pressure gradients that turbines with blades do. The flow from them is very smooth and even.”
Without a way to economically manufacture these giant disks, it became a creative dead end for Tesla. But Lo imagined that a micro version of these Tesla rotors might be exactly what he needed to make his experiment work. With the 3D printers he had in his lab, he started making Tesla rotors as small as a dime and deploying them as tiny pumps. When he spun them up, they indeed turned out a smooth, slow, steady flow — exactly what you find in the body’s tiniest blood vessels.
With a functional lab model now ready for action, Lo is set to fire up a series of experiments when UM-Dearborn’s labs reopen. In particular, by building on his previous work, he plans to imbed actual beta cells in tiny microfluidic systems to observe the impacts of fluids of varying viscosities. “For example, if greater shear in these pathways causes a stress response in the beta cells, or impacts their insulin production in some way, that would be an interesting result. But we have to run the experiment and see what happens.”
It’s one of those small breakthroughs that could help generate larger breakthroughs down the road. Right now, with so little known about the actual mechanics of how diabetes damages the body, the most potent tools doctors have for helping patients with advanced disease are largely behavioral. No doubt, prescribing a healthy diet and more exercise can be quite effective. But in practice, a variety of psychological and socioeconomic factors often make these treatments tricky for patients. If doctors some day had additional clinical tools that were informed by the biophysics of diabetes, it could fundamentally change the way we help millions of people living with the disease.