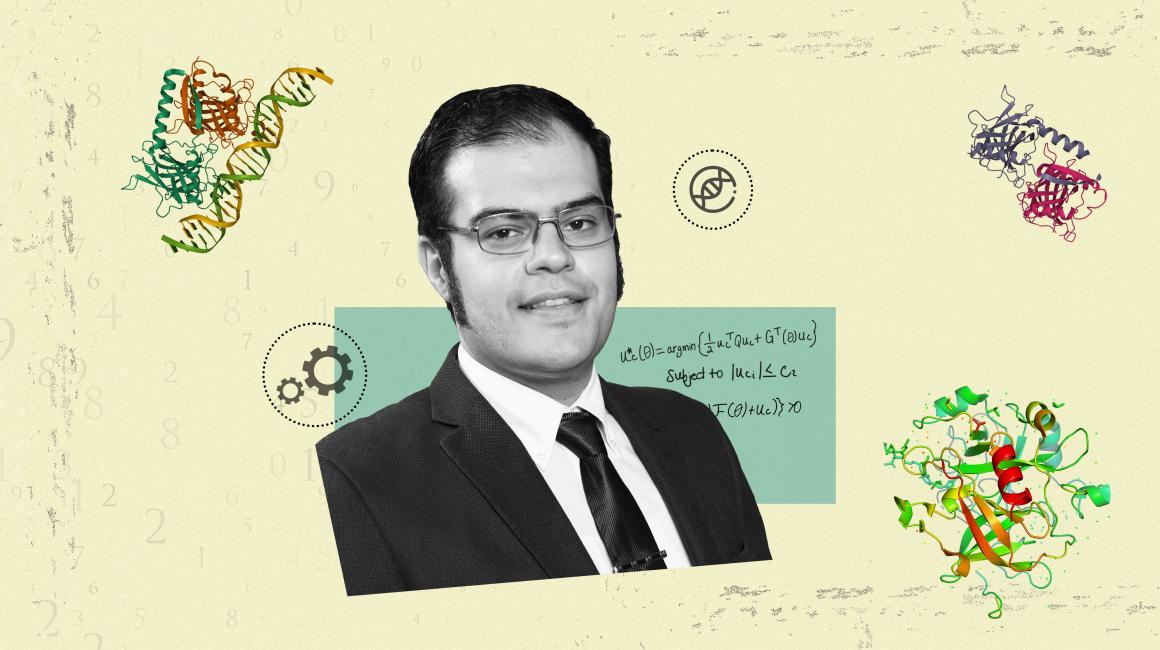
That robotics engineer Alireza Mohammadi would be fascinated by proteins shouldn’t be all that surprising. These complex organic molecules are truly the body’s tiny machines, whose intricate shapes and moving parts allow them to do all kinds of useful work, from delivering important messages to our cells to making our muscles move. In many ways, all the amazing work proteins do is still a great mystery to scientists. In fact, the universe of proteins is so vast and diverse, we still don’t even know exactly how many different kinds there are in our bodies. The current thinking is there are at least 20,000, all with distinct life-affirming jobs.
Like human-made machines, what a particular protein does depends a lot on its component parts and its overall shape. And also like machines, Mohammadi says proteins sort of have to be assembled. In their “unfolded” state, their linked individual amino acids resemble a long linear chain. But then, under very specific conditions, like the right temperatures, interatomic forces can bend, twist and fold these long chains into complex three-dimensional shapes. When they reach their final folded stage, proteins are ready to go to work in the body. How exactly proteins fold and unfold is a puzzle scientists would love to solve, and increasingly, engineers and computer scientists are getting involved in the effort. Mohammadi says one of the recent breakthroughs has come from artificial intelligence, which has given scientists the ability to predict the final shape of a protein based solely on the order of its amino acid chain sequence. “But these models can’t tell us how they evolve from their unfolded to folded states, and this is hugely important,” he says. “Consider a motor in a combustion engine — how does it generate work? It’s the predictable, back-and-forth motion of the piston inside a cylinder. So if we can understand — and ultimately predict — the motion of how proteins fold and unfold, we could potentially harness their work for useful purposes.” It could be years away, but he says the goal would be to “program” engineered proteins that would work like tiny robots inside the body to, say, deliver drugs or disrupt replicating viruses. The latest antiviral drugs being developed to treat COVID, for example, work by disrupting the coronavirus’ crucial spike protein.
Such a dream remains out of reach for now namely because how proteins move and evolve into their folded state is extremely complex, and Mohammadi says modeling such phenomena would be super computationally intensive. For example, in robotics, engineering the motion of a robot with even a few joints is already a sophisticated task. Mohammadi himself has even worked on snakelike robots with 10 linkages. Proteins, however, can have hundreds of linkages amongst their constituent amino acids, which twist, bend and combine in three-dimensional space. Needless to say, describing that motion would take some incredibly complicated mathematics. But as part of a new NSF-funded project, Mohammadi is taking a novel approach to tackling that challenge. His hypothesis is that the motion and path-planning algorithms that have been developed to govern the movement of robots might be just the ticket for describing how the body’s tiny machines also accomplish their work.
Over the next three years, Mohammadi and two biochemical researchers at the University of Texas at Dallas will aim to develop computational models that can accurately describe and predict proteins’ unique folding and unfolding behaviors. “On the one hand, our algorithms will have to be physically accurate, that is, they’ll have to describe what’s actually happening with the protein. But they also have to be computationally feasible. That is, your numerical algorithm can’t be so complicated that it blows up.” This work could be vital to helping other researchers develop protein-based treatments in the medical field. But interestingly, Mohammadi says it could also break open a new paradigm in robotics. He says for a long time, robotics engineering has relied on algorithms that are computationally “good enough” to do the kind of work that we’ve asked robots to do thus far. But we’ve known for a long time that more sophisticated intelligent robots capable of operating in congested environments — including self-driving cars — would need more perfect algorithms. It’s a similar challenge with protein folding, he says. “With protein molecules, in this higher-dimensional space, approximate solutions are not good enough,” he says. “So in developing algorithms that will solve that type of optimization problem for proteins, we could also be creating work that is useful for the robotics community. As our understanding of protein folding gets some inspiration from robotics, so too could proteins inspire a solution for a longstanding problem in robotics.”
###
Assistant Professor Alireza Mohammadi's collaborators on this NSF-funded project are Professor of Physics, Chemistry and Biochemistry Gerardo Andres Cisneros and Associate Professor of Biology Faruck Morcos from the University of Texas at Dallas. Story by Lou Blouin.